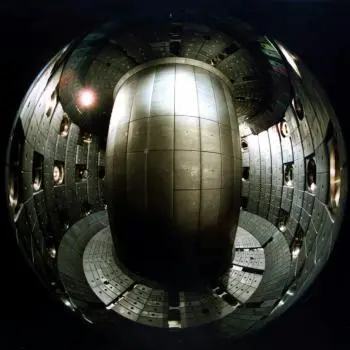
Nuclear fusion is the process by which atomic nuclei combine to form a heavier nucleus, releasing an enormous amount of energy in the process. This is the process that powers the stars, including our sun.
To carry out controlled nuclear fusion on Earth, extremely specific conditions are required due to the high temperatures and densities necessary to overcome the electrostatic repulsion between nuclei.
The main requirements to achieve controlled nuclear fusion are the following:
-
High temperature: An extremely high temperature needs to be reached, typically in the range of hundreds of millions of degrees Celsius. At these temperatures, the atoms ionize, forming a hot plasma.
-
Proper Density and Pressure: The plasma must be confined and compressed to increase the probability that the nuclei will collide and fuse.
-
Long Confinement Time: Nuclear fusion requires a long enough time for a significant number of fusion reactions to occur.
-
Magnetic or inertial confinement: There are two main approaches to confine plasma: magnetic confinement and inertial confinement. In magnetic confinement, the plasma is kept suspended and contained by magnetic fields. In inertial confinement, the plasma is compressed by shock waves generated by lasers or accelerated particles.
-
Suitable Fusion Fuel: The most common fuel for nuclear fusion is a mixture of hydrogen isotopes, such as deuterium and tritium.
-
Absence of Contaminants: Materials that come into contact with the plasma should be minimized as they could contaminate the fusion process and cool the plasma.
Plasma confinement strategies
There are various containment strategies used in nuclear fusion experiments to keep the plasma in a condition suitable for fusion to occur. Here are some of the top two strategies:
Magnetic confinement
The tokamak and the stellarator are two types of experimental devices used to investigate and achieve magnetic confinement of plasma. Both devices are designed to confine and heat plasma to the high temperatures necessary for nuclear fusion to occur.
The tokamak uses magnetic coils to generate a toroidal magnetic field that confines the plasma in a ring. The magnetic field prevents the plasma from escaping and scattering, keeping it in a hot, confined state.
The stellarator, unlike the tokamak, uses more complex magnetic coils to create a three-dimensional magnetic field without the need for electrical currents in the plasma. This allows the plasma to remain stable without the need for heating currents.
Inertial confinement
inertial confinement focuses on compressing and heating the fusion plasma using shock waves or mechanical compression.
The basic principle of inertial confinement is to subject the fusion fuel (usually a mixture of hydrogen isotopes, such as deuterium and tritium) to high pressures and temperatures so that the atomic nuclei collide with enough kinetic energy to overcome the electrostatic repulsion and fuse.
There are two main approaches to achieving inertial confinement:
-
Direct Compression Fusion: In this approach, a small capsule of fusion fuel is compressed quickly and in a controlled manner using shock waves generated by high-powered laser beams or accelerated particles. The compression of the fuel increases the density and temperature of the plasma in the core of the capsule, favoring nuclear fusion. This concept is known as "inertial confinement fusion by implosion".
-
Magneto-inertial confinement fusion: This strategy combines aspects of magnetic and inertial confinement. A device that combines inertial plasma compression with magnetic confinement is used to extend plasma lifetime and improve fusion efficiency. An example of this is the concept of "magneto-inertial fusion" (MIF), which seeks to take advantage of the best of both approaches to achieve nuclear fusion.
Nuclear fusion research reactors
Scientists are working with research reactors to find ways to overcome the technical limitations of nuclear fusion.
Some of the most prominent research reactors in the field of nuclear fusion are as follows:
ITER (International Thermonuclear Experimental Reactor)
It is one of the largest and most ambitious projects in the field of nuclear fusion. It is located in Cadarache, France, and is an international collaboration between 35 countries. ITER is a tokamak that aims to demonstrate the scientific and technological feasibility of nuclear fusion as a sustainable energy source without carbon emissions. ITER is expected to reach the "ignition" point, at which the energy released by fusion reactions exceeds the energy needed to heat and confine the plasma.
JET (Joint European Torus)
Located in the UK, JET is the largest tokamak in operation and has been a pioneer in nuclear fusion research since its inception in 1983. It is a collaboration between the European Union and other partner countries. JET has done important research on magnetic confinement and plasma heating.
EAST (Experimental Advanced Superconducting Tokamak)
EAST is located in China and is one of the largest and most advanced tokamaks in the world. He has conducted research in superconductivity and plasma heating technologies.
Wendelstein 7-X
It is a stellarator located in Germany. It differs from other stellarators by its optimized magnetic field design to improve plasma confinement. Wendelstein 7-X is the largest and most advanced stellarator in operation and has conducted research to improve plasma stability and magnetic confinement.