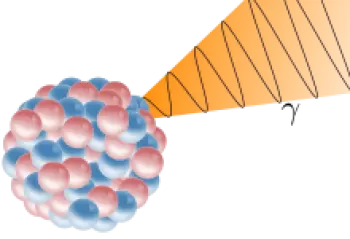
In nuclear physics, gamma rays are the electromagnetic radiation produced by the radioactive decay of atomic nuclei. The lowercase Greek letter γ often symbolizes them.
Other types of radiation in the electromagnetic spectrum are X-rays, visible light, and ultraviolet (UV) radiation. The only difference between them is the photon energy.
Photons are elemental subatomic particles carriers for all forms of electromagnetic radiation.
Gamma radiation is one of the most dangerous types of very high-frequency radiation for humans, as are all ionizing radiation. The danger stems from the fact that they are high-energy waves capable of producing molecular changes and damaging the molecules that make up cells. These transformations can cause genetic mutations, cancer cells, or even death.
Where do gamma rays come from?
Generally, they are associated with nuclear power, nuclear reactors, and nuclear explosions. However, radioactivity is found in our natural environment, from cosmic rays, which bombard us from the sun and galaxies outside our solar system to some radioactive isotopes that are part of our natural environment.
In general, gamma rays produced in space do not reach the Earth's surface, as they are absorbed in the upper atmosphere. To observe the universe at these frequencies, it is necessary to use high-altitude balloons or space observatories.
In both cases, the Compton effect is used to detect gamma rays. These gamma rays are produced in high-energy astrophysical phenomena such as supernova explosions or active galactic nuclei.
On Earth, we can observe natural sources of gamma rays both in the decay of radionuclides and in the interactions of cosmic rays with the atmosphere.
In astrophysics, GRB (Gamma Ray Bursts) are called gamma-ray sources that last a few seconds or a few hours, followed by a decreasing brightness of the X-ray source for a few days. They occur randomly in the sky, and their origin is still under scientific discussion. In any case, they seem to constitute the most energetic phenomena in the Universe.
What are the uses of gamma rays?
A wide variety of applications use sources of gamma radiation. Here are some examples:
-
In the industry for the sterilization of materials in irradiation plants.
-
Medical applications of treatment and diagnosis to destroy cancer cells, for example. This form of radiation is also used to sterilize medical and veterinary products.
-
Food preservation treatments such as pasteurization and disinfection.
-
Pharmaceutical products.
-
Manufacture of cosmetics.
-
Treatment of hospital waste.
Characteristics of gamma rays
Gamma rays are more penetrating than radiation from other forms of radioactive decay (alpha or beta radiation) due to the lower tendency to interact with matter.
Gamma radiation is made up of photons: this is a substantial difference from alpha particles, helium nuclei, and beta radiation, which is made up of electrons. Gamma-ray photons, not being endowed with mass, are less ionizing. At these frequencies, the description of the phenomena of interactions between the electromagnetic field and matter cannot ignore quantum mechanics.
What is the difference between gamma rays and X-rays?
Gamma rays are distinguished from X-rays by their origin: they are produced by nuclear or atomic transitions. On the other hand, Energy transitions make X-rays due to electrons entering external quantized energy levels from external quantized energy levels.
How gamma radiation interacts with matter?
When a gamma-ray passes through matter, the chances of absorption in a thin layer are proportional to the thinness of that layer, leading to an exponential decrease in intensity.
When passing through matter, gamma radiation primarily ionizes in three ways:
1. Photoelectric Effect
It describes when a gamma photon interacts with an atomic electron and transfers its energy to it, ejecting said electron from the atom. The kinetic energy of the resulting photoelectron is equal to the incident high-energy photons minus the electron's binding energy.
This effect is the dominant energy transfer mechanism for x-rays and gamma-ray photons with energies below 50 keV, but it is less crucial at higher energies.
2. Compton Effect
It refers to the interaction where an incident gamma photon makes an atomic electron gain enough energy to cause its ejection. With the remaining energy of the original photon, a new low-energy gamma photon is emitted with a different emission direction than the incident gamma photon.
The probability of the Compton Effect decreases as the photon energy increases.
The Compton Effect is considered to be the primary mechanism of gamma-ray absorption in the intermediate energy range between 100 keV to 10 MeV, an energy range that includes most of the gamma radiation present in a nuclear explosion. The Compton effect is relatively independent of the atomic number of the absorbing material.
Pair creation
Due to the Coulomb force's interaction in the nucleus's vicinity, the energy of the incident photon is converted into the mass of an electron-positron pair. A positron is the antiparticle equivalent to an electron; it has the same mass as an electron, but it has a positive charge of equal strength to the negative charge of an electron.
The energy above the rest mass equivalent of the two particles (1.02 MeV) appears as the kinetic energy of the pair and the nucleus. The positron has a concise life (about 10 –8 seconds). At the end of its period, it combines with a free electron. The entire mass of these two particles is then converted into two gamma photons of 0.51 MeV energy each.
What can stop gamma radiation?
γ-ray shielding requires much thicker materials than those needed to shield alpha (α) and beta (β) particles, which can be blocked by a simple sheet of paper or a thin metal plate. The energy of gamma rays has a significant penetrating power because of its shortest wavelength.
Materials with a high atomic number and high density have a greater ability to absorb gamma radiation.
Shielding materials are generally measured based on the thickness required to halve radiation intensity. Obviously, the higher the photon energy, the thicker the shielding necessary must be.
In nuclear power plants, steel and cement are used to protect the containment vessel from gamma particles. In addition, the water protects against radiation produced during the storage of nuclear fuel rods or the transportation of the nuclear reactor core.