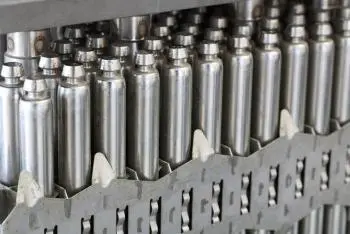
Control rods in nuclear power plants are key components for regulating reactor power and ensuring its safety.
These devices, made of highly efficient neutron-absorbing materials, play a crucial role in maintaining the balance of the nuclear reactions occurring in the reactor core.
Without them, chain reactions could become unstable, increasing the risk of overheating and possible accidents.
Nuclear chain reaction
A nuclear chain reaction occurs when a neutron hits an atom of a fissile material, such as uranium-235 or plutonium-239, splitting it into smaller fragments and releasing a large amount of energy.
This process generates new neutrons that can hit other atoms, starting a repeating cycle. The speed of this reaction depends on the number of neutrons available and how they interact with the nuclear fuel.
Operation of control rods
The control rods are designed to manage this chain reaction by absorbing neutrons.
They are made from materials such as boron carbide or alloys of silver, indium and cadmium, which have a high capacity to capture neutrons. Their cylindrical design and dimensions, similar to those of nuclear fuel rods, allow them to be inserted directly into the reactor core.
The further the control rods are pushed into the core, the more neutrons they absorb. This reduces the number of neutrons available to trigger new fission reactions, thereby decreasing the reactor's power. Conversely, removing them increases the number of active neutrons, increasing power.
Reactor power regulation
The regulation of a nuclear reactor is a complex process involving several systems, with the control rods being one of the most important. These rods allow the frequency of nuclear reactions to be adjusted in real time and are also essential during emergencies.
Quick stops or "scram"
In critical situations, such as an unexpected power surge or a cooling system failure, control rods are used to quickly stop the chain reaction.
In pressurized water reactors (PWRs), the rods typically hang in the reactor and are supported by electromagnets. If a power loss occurs, the electromagnets are deactivated and the rods fall by gravity into the core, stopping the reaction. This method is common, though not universal, in PWR designs.
In boiling water reactors (BWR), control rods are introduced from the bottom of the reactor using hydraulic or mechanical systems. This design ensures redundant and reliable control, as it does not rely solely on gravity.
Although the principle is different, the goal remains the same: to stop the nuclear chain reaction quickly and safely.
Additional factors in reactor control
Reactor performance is also influenced by temperature, coolant flow, and the accumulation of fission waste products.
Some of these products, such as xenon-135, have a high capacity to absorb neutrons. The accumulation of xenon-135, known as "xenon poisoning," can stabilize or even temporarily shut down a reactor after a significant power reduction, requiring constant adjustments to the position of the control rods.
Safety and cooling after a stop
When the control rods are fully inserted into the core, the nuclear chain reaction stops and the reactor enters a subcritical state. However, the radioactive material in the core continues to generate residual heat due to the radioactive decay process, known as decay heat.
This heat represents a significant percentage of the initial thermal power and must be dissipated in a controlled manner to avoid overheating that could damage the reactor or cause a serious accident.
Secondary cooling systems are essential to this process. Without them, even with the control rods fully inserted, a core meltdown could occur, as occurred in the Fukushima and Chernobyl accidents.
Relationship between control rods and nuclear accidents
The safety of nuclear power plants depends largely on the correct functioning of the control rods, but these are not the only factor.
Historically, failures in complementary systems and human error have been the main causes of catastrophic nuclear accidents.
The Chernobyl accident
The Chernobyl disaster in 1986 is a tragic example of how faulty control rod design and poor operational management can lead to devastating consequences.
In that case, the control rods had graphite tips, a material that initially increased reactivity rather than decreased it. This effect occurred during the initial insertion of the rods, due to the specific design of the RBMK reactor.
During a poorly planned test, this defect, combined with human error, failures in other systems and the lack of adequate containment, led to an explosion and the release of large quantities of radioactive material.
Prevention of criticality accidents
In other accidents, neutron-absorbing materials such as boron or cadmium have been used to mitigate criticality situations.
For example, in carbon dioxide-cooled reactors, nitrogen gas can be injected into the primary circuit to absorb neutrons and stop the chain reaction if the control rods are not sufficient.
The use of these materials has also proven effective in reducing the activation of materials surrounding the core, thus minimizing the risks of radioactive contamination in the event of an emergency.
Innovations and advances in control rods
Nuclear technology continues to evolve, and control rods are no exception. Researchers are constantly working on improving materials and designs to make them more effective and reliable.
Some of the latest innovations include:
- Advanced Materials: New compounds based on boron carbide and rare earth alloys are being developed to increase neutron absorption capacity and improve wear resistance.
- Integrated sensors: Some modern control rods incorporate sensors that allow real-time monitoring of their position and the level of neutrons they are absorbing. This facilitates more precise control of the reactor.
- Faster Insertion Systems: Improved designs in insertion and extraction mechanisms allow for faster responses during emergencies.