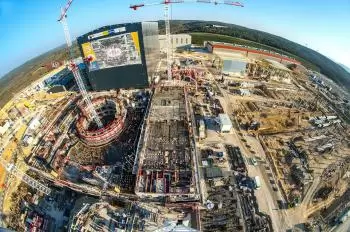
The ITER (International Thermonuclear Experimental Reactor) project is one of the most ambitious international efforts in the search for a clean and practically inexhaustible source of energy: nuclear fusion.
Located in Cadarache, in the south of France, this experiment seeks to demonstrate the technical and scientific feasibility of fusion as an alternative to current energy sources.
What is nuclear fusion?
Nuclear fusion is the process that powers the Sun and other stars. It involves the union of two light atomic nuclei to form a heavier one, releasing an enormous amount of energy in the process.
Unlike nuclear fission, which produces long-lived radioactive waste, fusion promises to be a much cleaner source of energy. Moreover, the elements needed for fusion, such as deuterium and tritium, are either abundant or can be produced relatively easily.
Fusion not only promises low carbon emissions, but also global energy stability.
Deuterium can be extracted from seawater, while tritium can be generated within the reactor itself using lithium. This makes fusion a potentially sustainable energy option for future generations.
The objectives of ITER
ITER's main goal is to demonstrate that it is possible to generate more energy than is consumed in the fusion process. In technical terms, this is known as achieving an energy "gain."
The ITER reactor is designed to produce 500 megawatts of fusion power with an input of 50 megawatts, thus achieving a tenfold increase in efficiency.
ITER also has other important goals, such as developing materials that can withstand the extreme conditions inside a fusion reactor and testing technologies for safe fuel handling. This knowledge will be key to the design of future commercial reactors.
The challenges also include perfecting heating systems and precise plasma control under extreme conditions.
How ITER works
The heart of ITER is a device called a tokamak, a doughnut-shaped reactor that uses powerful magnetic fields to confine plasma, a state of matter at extremely high temperatures.
For fusion to occur, the plasma must reach temperatures of about 150 million degrees Celsius, ten times hotter than the Sun's core. At these temperatures, the deuterium and tritium nuclei have enough energy to overcome their electrical repulsions and fuse.
Magnetic confinement is essential to keep the plasma stable long enough for fusion to occur.
At ITER, this is achieved with a complex system of superconducting magnets that generate intense and precise magnetic fields. These magnets are cooled to temperatures close to absolute zero (-269 °C) using liquid helium, which makes it possible to handle the large amounts of energy generated.
Once the plasma is confined, advanced techniques are used to heat it to the required temperatures. These include radiofrequency heating and the use of high-energy neutron beams to increase the temperature of the plasma.
An international effort
ITER is a truly global project. Thirty-five countries are involved, including members of the European Union, the United States, Russia, China, India, Japan and South Korea. Each contributes funding, technology and key components for the reactor.
This collaboration not only strengthens scientific exchange, but also symbolizes a joint effort to address the energy challenges of the future.
The ITER collaboration model also encourages the development of technological capabilities in participating countries.
Many nations have created specialized industries to manufacture the complex components required by the tokamak, which is driving advances in areas such as superconductivity, robotics and materials engineering.
The challenges of the project
Although the promise of nuclear fusion is impressive, ITER faces significant technical and financial challenges.
Building a tokamak of this magnitude is an extremely complex task, and project costs have increased considerably since its inception. Originally budgeted at around €5 billion, the current cost is estimated at over €20 billion.
Technical challenges include developing materials capable of withstanding the intense radiation and heat generated inside the reactor. In addition, the integration of components, which come from different countries, requires careful coordination to ensure that everything works harmoniously.
On the other hand, there is also uncertainty about the behavior of plasma under such extreme conditions.
Although previous simulations and experiments have been performed on other, smaller tokamaks, ITER represents an unprecedented leap in scale and complexity.
When will we see results?
ITER is expected to begin its first plasma tests in the 2030s.
This milestone will be key to assessing whether the reactor design can meet its energy goals. If successful, ITER will be the precursor to commercial fusion reactors that could be operational by the mid-21st century.
The project schedule has been subject to delays due to its complexity and the impact of global factors, such as the COVID-19 pandemic. However, the progress made so far is promising and reflects a joint effort by all participants.
The promise of fusion
If ITER meets its goals, it could mark the beginning of a new energy era. Fusion has the potential to provide an almost unlimited source of energy, with low carbon emissions and without the long-term radioactive waste problems associated with nuclear fission.
In addition, fusion energy could significantly contribute to reducing dependence on fossil fuels and mitigating climate change.
The scientific community believes that the success of ITER will also inspire further developments in other areas. Technologies developed for the reactor could have applications in medicine, transport and space exploration.